As the world oil situation becomes a larger economic focus for billions of global consumers, the CHORUS University of Calgary research project has expanded its integrated science international industry research team and heavy oil international sponsor focus to address these needs. Global energy consumption is on the rise; however, conventional oil reserves continue to decline. As a result, greater emphasis is being placed on maximizing production methods for heavy oil and bitumen reserves. Thermal recovery methods have been utilized to unlock both types of reserves. But with rising prices, producers are seeking alternative production methods that will minimize their operating costs. Cold production is a cost-effective production method and is gaining ground as a viable means to produce heavy oil. The CHORUS heavy oil industry sponsors now include the major heavy oil industry field companies from Canada, Alaska, Venezuela, Europe, Russia, Kuwait, Iran and China to address this global oil energy need. The principal objective of CHORUS is to set up a working research plan with the global heavy oil industry, to integrate the various industry and university reservoir characterization, advanced rock physics, geophysical, geosciences and engineering simulation techniques that are crucial to heavy oil exploration, development and the successful recovery methods of the new more economic methods of heavy oil cold production processes. The initial and main focus of the consortium is on cold production, with its wormholes and foamy oil behavior.
In the cold production project, CHORUS is using seismic attributes as indicators of reservoir changes caused by cold production. Preliminary results show substantial promise in detecting changes in gas saturation and some possibility of detecting wormholes. One of CHORUS’ main goals is to integrate time-lapse seismology, seismic modeling, and reservoir characterization. Rock physics provides a crucial link between reservoir characterization and time-lapse seismology. CHORUS is collaborating with rock physics groups at the University of Alberta and Core Lab. However with rising prices, producers are seeking alternative production methods that will minimize their operating costs. Cold production is a cost-effective production method and is gaining ground as a viable means to produce heavy oil. Cold production is the most cost-effective production method already being utilized in Canada and is gaining ground as a viable means to produce heavy oil due to the growing use of progressing cavity pumps (PCP). These pumps act like augers and suck both sand and oil into the well. With no natural gas required like with thermal methods, the biggest benefit of cold production is that it provides producers with energy savings. At the onset of cold production, the PCPs produce about 60 percent oil and 40 percent sand. Within about three months, these ratios improve to about 95 percent oil. These production improvements are attributed to the development of high porosity tubes known as “wormholes”.
These wormholes tend to spread horizontally creating a network of wells without having to use a drilling rig. With cold production, after the heavy oil is brought to the surface by the PCPs, a settling tank then separates the oil and sand. Trucks would then routinely visit the production site to remove the produced oil and clean sand from the tanks. The CHORUS group has been involved recently with the seismic monitoring of cold production processes. The correlation between the seismic anomalies and the reservoir production data in cold production fields is very compelling. To better understand these correlations and the implications for enhanced oil recovery, CHORUS has directed its project research efforts towards the integrated science studies of geochemical, geological, geophysical and reservoir production data. This research is leading toward a greater understanding of reservoir properties and should enhance production from heavy oil fields.
Please contact the CHORUS Project Manager at jembleto@ucalgary.ca for more consortium information.
Research Project Advances
The CHORUS integrated science group, has been responsible for the characterization of fluid properties and oil composition data for the Nexen Plover Lake cold production project. CHORUS has synthesized a large array of data from core sampling, organic chemistry and viscosity lab-work, and statistical analysis. This data has been integrated via engineering software and a numerical simulator, resulting in reservoir engineering models. CHORUS has a project vision for how this emerging field can change the industry. “With the integration of geochemistry, geophysics, geomechanics and reservoir engineering within the CHORUS/Nexen Plover Lake project, we can begin to investigate the processes that control the dynamic production footprints and fluid physics in heavy oil reservoirs, and history-match the effects of CHOPS on wormhole propagation. The Plover Lake project has been greatly aided by Schlumberger’s Petrel and CMG’s Stars software, which enable the incorporation of large amounts of data into physical models. These models enable us to pull apart the processes that dominate in the subsurface, which is a non-trivial exercise. The CHORUS group is at a critical stage in our modeling, and we are working hard to complete this project.” Future research will involve applications for the enhanced oil recovery (EOR) solvent-based processes, such as VAPEX, asphaltene precipitation and new tar solvents, reservoir characterization field data works that include the seismic monitoring geochemical/reservoir modeling and simulation. CHORUS has contributed to many research papers and technical journal reports have been published in several of the national and international geological, geophysics, geochemical and reservoir engineering journals. Also, CHORUS has presented several integrated science results at the 2008 annual industry sponsors technical meeting, individual oil industry client private meetings and presentations have been made at many national and international heavy oil meetings, workshops and other Canadian oil industry symposia. Several of these integrated science papers and presentations have received both national and international recognition, as well as awards from industry and professional scientific organizations and societies. The high quality of the CHORUS project and its research personnel are indicated by a number of Canadian and international awards. CHORUS is now recognized internationally as the leading heavy oil cold production reservoir characterization and seismic integrated research technology.
One of the challenges that oil and gas industries are dealing with now is to know the variations in the viscosity in reservoir scale. Viscoelastic seismic monitoring can be used for the purpose of viscosity estimation for such a problem. Viscoelastic behaviour is a time dependent, mechanical non instantaneous response of a material body to variations of applied stress (Carcione, 2007). That means the response of the medium to stress is not indefinite and occurs with a delay due to the viscous behaviour of the material. It is said that the material has memory. It is said that the material has the memory. A good example of a viscoelastic response is the suspension system of a vehicle. There is a spring which acts as the elastic part and a dashpot which absorbs the shock and delays the elastic response.
To formulate the viscoelastic behaviour, rock physicists have used springs and dashpots as the components of viscoelasticity. There are different mechanical models for deriving the stress-strain relationship.
This model is of more interest to us since it better represents the earth material. In order to generalize this model, one can connect multiple Zener components in parallel to form the “generalized Zener model.”
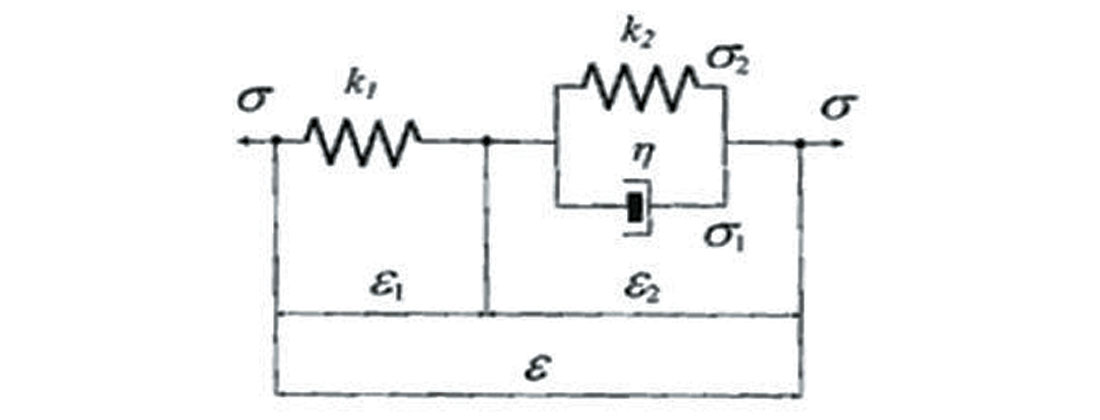
The following equations show the relationship between quality factor (inverse attenuation), frequency and viscosity which are shown by Q, ω and η, respectively.

Where, in the equation above, we have:

where k1 and k2 are spring constants.
The following figure shows the theoretical variations in Q with viscosity.
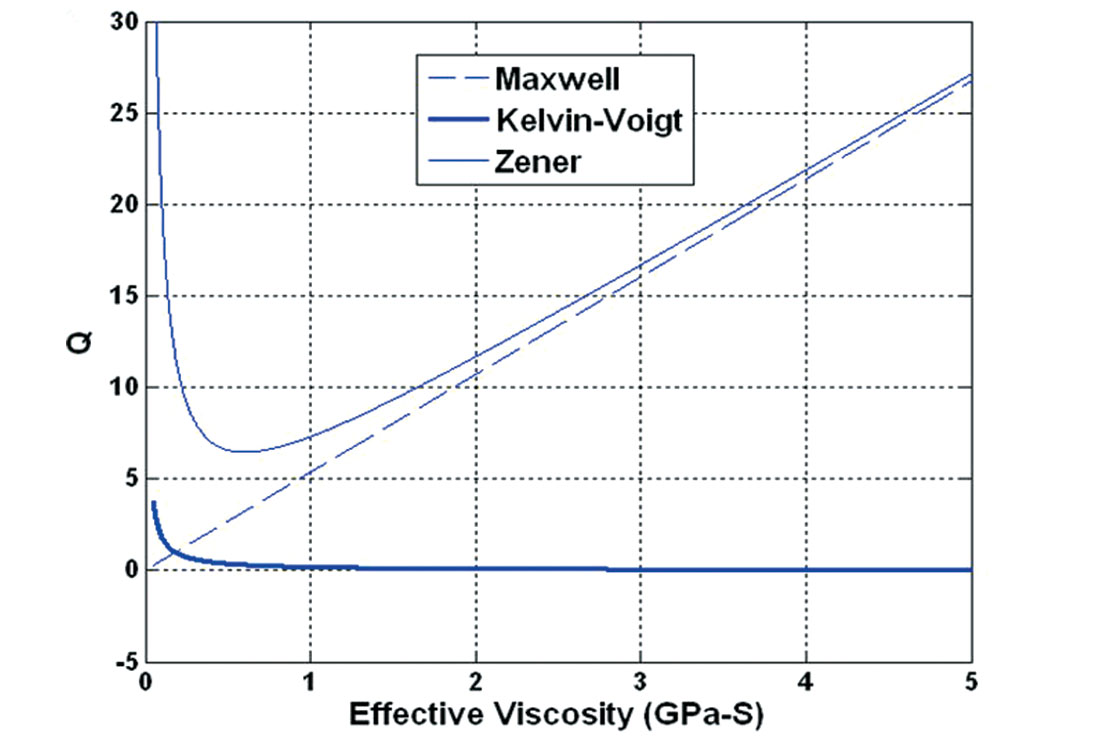
Understanding the effects of viscosity on seismic response will give us the tool for heavy oil reservoir monitoring. This is a work in progress and researchers in CHORUS are working towards a comprehensive approach to heavy oil reservoir characterization.
The direct relationship between viscosity and the attenuation of seismic waves is also shown in experimental results. Recent experiments relating Q to viscosity (and temperature) have been conducted by CHORUS’s Doug Schmitt at the University of Alberta and by Mike Batzle’s group at the Colorado School of Mines. Both theory and experiment show an initial increase in attenuation with temperature as heavy oil goes from “glass stage” to a viscous fluid, followed by a decrease in attenuation as the heavy oil is heated to a less viscous fluid.
All interpretations of seismic data involve the analysis of seismic displays. In his recent PhD thesis, Steve Lynch, a CHORUS researcher, has developed new and innovative techniques for displaying seismic data. Lynch’s thesis contrasts the conventional variable area wiggle seismic trace display (shown in Figure 3) with a display known as Seiscape (shown in Figure 4). The Seiscape display (first published by Lynch in 2000) shows seismic amplitudes as hills and valleys. Seiscape and other seismic displays are described in Lynch’s pioneering PhD thesis at the University of Calgary entitled More than meets the eye – A study in seismic visualization. It is believed that this research will cause seismologists to re-think their methods of seismic trace displays and will aid interpretation.
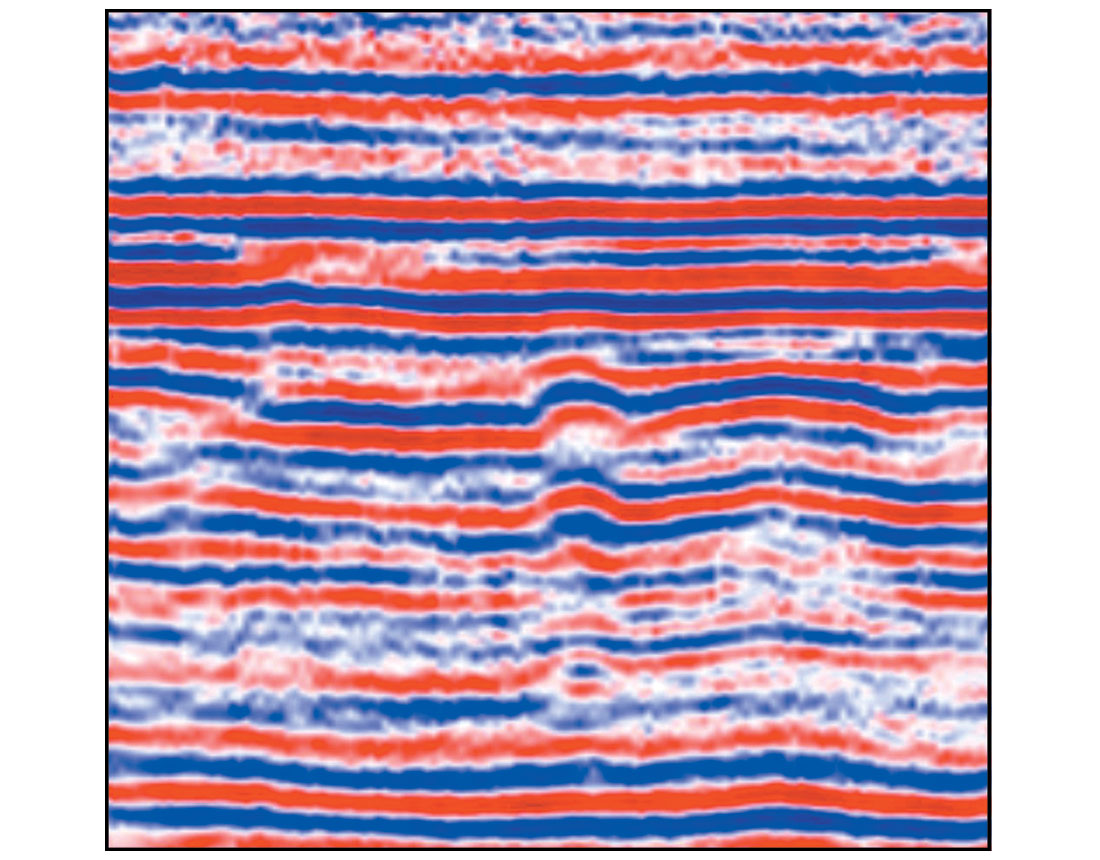
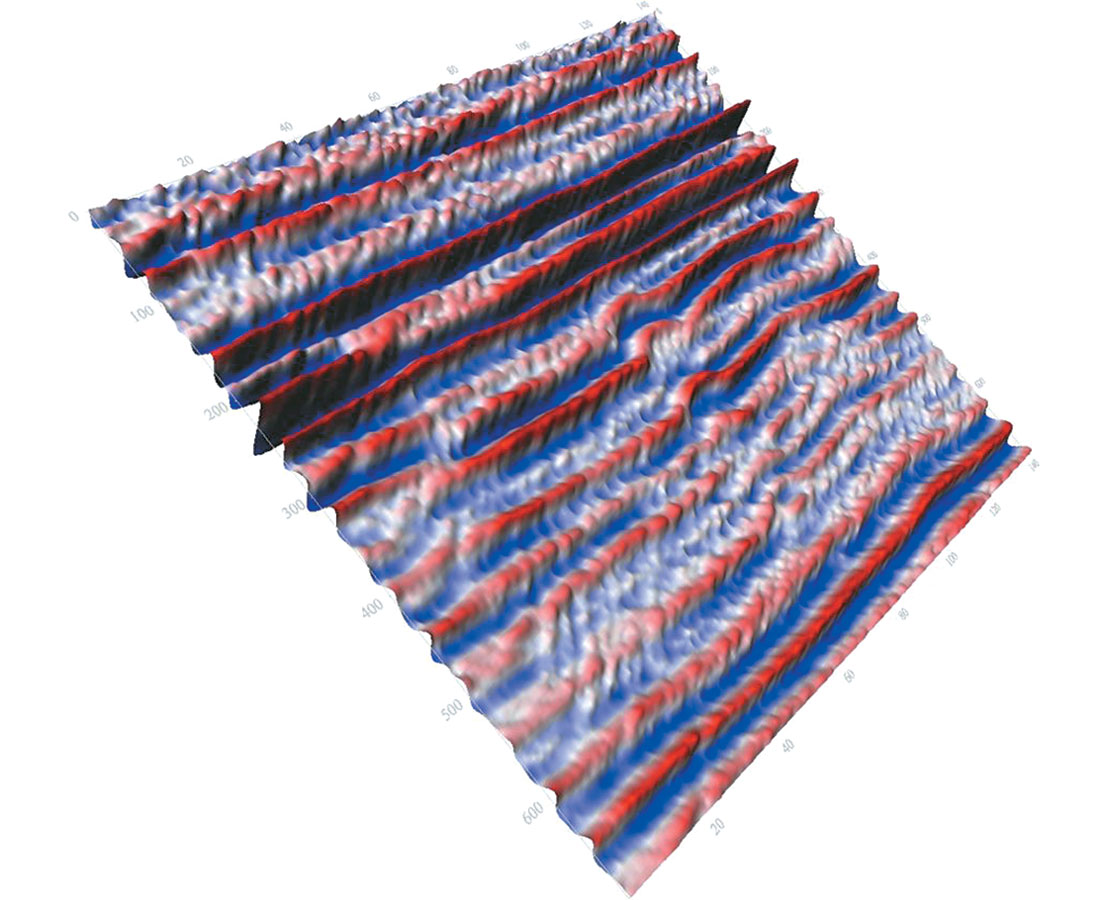
While this seismic display technology has wide-ranging applications to any number of seismic interpretations, it is especially of interest to those involved in seismic analysis of heavy oil fields where resolution of thin sandstone and shale layers is a key challenge in interpretation. As pointed out by Lynch (2008), seismic visualization is a key part of the seismic resolution challenge.
Ultimately, one of the main goals of reservoir geophysics is to incorporate geophysical information into reservoir characterization models. Akey link between the seismic velocity information and reservoir models is provided by rock physics. Traditionally, we have used Gassmann’s equation which relates the pore fluids and rock matrix to seismic velocities. It predicts the bulk modulus of a fluid-saturated porous medium using the known bulk moduli of the solid matrix, the frame and the pore fluid by following:

where K* , Kd , Km , Kf , and ϕ are the saturated porous rock bulk modulus, the frame rock bulk modulus, the matrix bulk modulus, the fluid bulk modulus and the porosity. It is assumed that the shear modulus μ* of the saturated rock is not affected by fluid saturation, so that

with μd being the dry frame shear modulus.
The P-wave and S-wave velocities, Vp and Vs , for an isotropic, homogeneous, elastic material are given by

and

where ρ* is the saturated rock bulk density and can be calculated as

where ρm and ρf are the densities of solid grains and the fluid mixture at reservoir conditions.
Equations (2) to (6) establish the relationships between the rock moduli and the seismic velocities. There are several assumptions regarding the accuracy of the Gassmann’s equation for calculating the seismic velocities in the reservoir type under consideration (ref. Han and Batzle, 2004). One of them is that the pores are filled with a frictionless fluid (liquid, gas, or mixture). This assumption implies that the viscosity of the saturating fluid is zero. This may be the most questionable assumption for heavy oil, especially at cold temperatures (about 20-40 ºC).
Relationships between seismic velocity and reservoir model parameters will allow us to enhance reservoir modeling. Reservoir models for cold production of heavy oil (from the research of Hossein Aghabarati, a CHORUS Ph.D. grad student) are shown in Figure 5. The reservoir model prior to cold production, as shown on the left, shows a random distribution of p o rosities with most cells having 20-40% porosity. Following 10 years of cold production for a well at the center of the model, we see extremely high porosities exceeding 60% emanating out f rom the well, as shown on the right side of Figure 8. The map of porosities is highest at the well location, but does show pre ferential branching directions. These preferential directions can be determined by time-lapse seismology, as shown by data examples in Lines et al. (2003) and Zou et al. (2004).
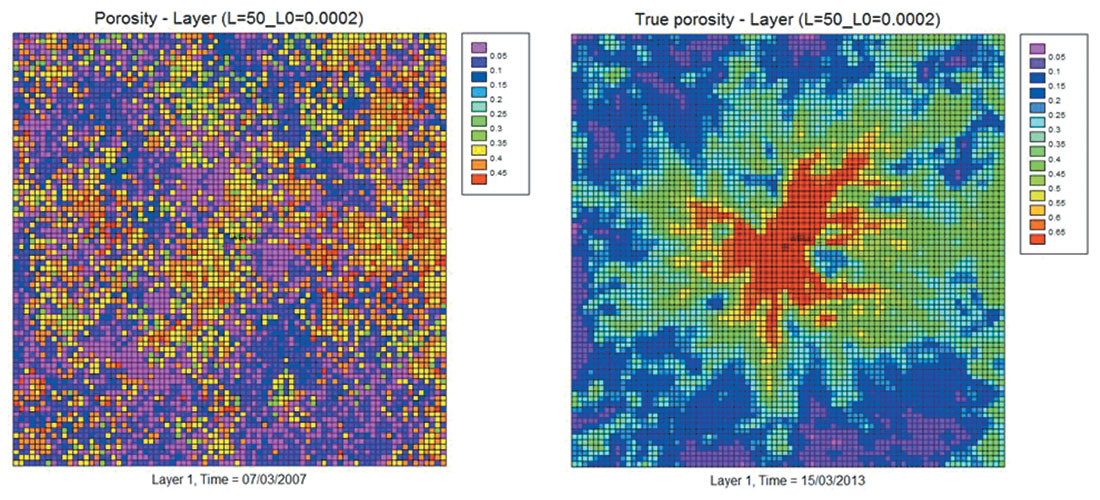
The goal of seismic time-lapse surveys would be to correct or improve these reservoir models in cold production. Our experience has shown that time-lapse seismology, rock physics can aid in the reservoir characterization of porosities. Our next immediate goal will be to utilize seismic and rock physics data to characterize reservoir fluids, in particular, the heavy oil viscosity.
As far as the global integrated heavy oil research science needs are concerned, CHORUS is listening to the world oil industry and is moving forward in a very positive path. Cold production is becoming a very new but well known successful process of in the area of heavy oil recovery. CHORUS is currently developing several new CHOPS technical integrated science methods of enhanced oil recovery processes, and new geoscience/engineering coding for the many difficult integrated reservoir characterization research projects around the world. CHORUS is also directly collaborating/working with the major international heavy oil service companies such as Schlumberg e r, CGG/Veritas, BGP and Fugro-Jason.
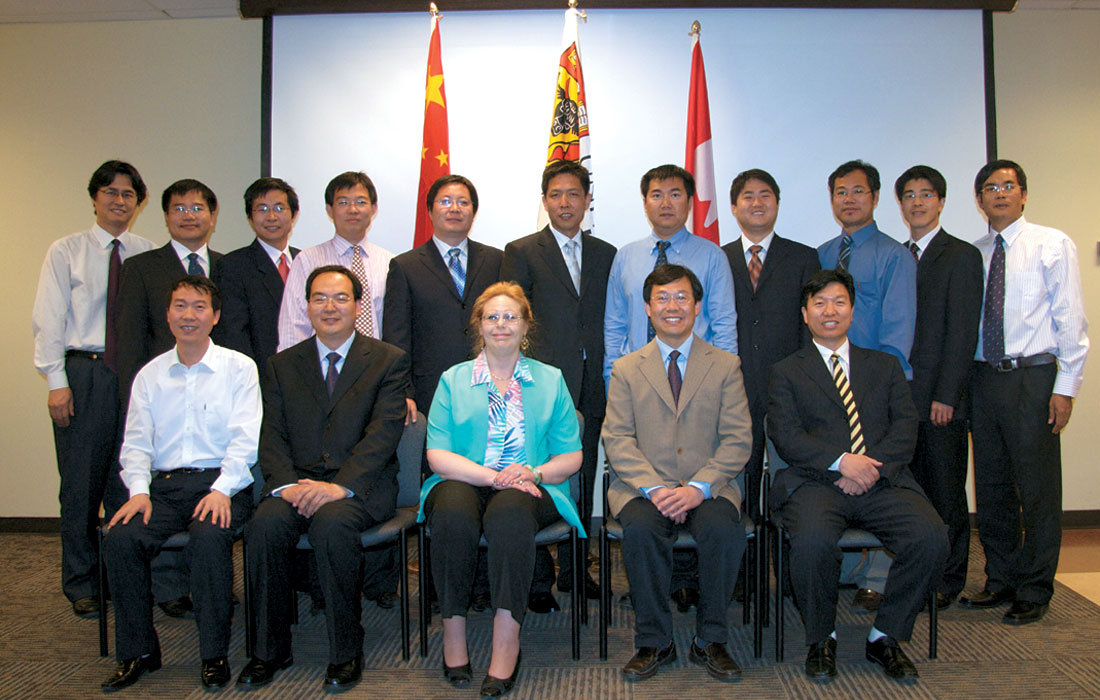
CHORUS will be holding its next heavy oil technical sponsors meeting in the early portion of 2009 due to the many new and exciting technical research results. Please contact Joan directly at jembleto@calgary.ca for any further heavy oil research questions or interest in becoming a sponsor member of the CHORUS Heavy Oil Integrated Science Project located at the U of C Geoscience Department.
Join the Conversation
Interested in starting, or contributing to a conversation about an article or issue of the RECORDER? Join our CSEG LinkedIn Group.
Share This Article