The word “geophysics” in oil exploration is often used synonymously with “seismic”, overlooking many other fruitful techniques. In mineral exploration and engineering projects, applicability of seismic imaging is often limited and other geophysical methods take the front seat.
Gravity and magnetic methods, which are discussed in this article, are extremely useful in both mineral and oil exploration. Unfortunately, among oil-industry geophysicists and managers the knowledge and appreciation of these techniques tend to be comparatively thin. Rooted in over-specialized college training, a too-narrow focus on only some geophysical methods impoverishes oil exploration if potential- field surveys are underutilized. By limiting geophysicists’ ability to switch between oil and mining industries, it restricts their employment flexibility and career choices.
The multi-faceted exploration market calls for many different types of geophysical work. Professional associations and alumni groups need to put pressure on academic institutions to diversify and round out their curricula. Fluctuating economic conditions, and future well-being of new graduates, demand nothing less.
A few basics
Gravity and magnetic geophysical methods are passive. They rely on no controlled sources but seek out naturally occurring variations in the earth’s gravity and magnetic fields. For this reason, some military uses of these surveys have long included quiet detection of submarines and volatile unexploded munitions.
Metamorphosed, igneous and polydeformed rocks, which are common subjects of mineral exploration, can be far more complicated than the stratified rocks examined in the search for oil and gas. Hard-rock geology presents an explorer with a mind-boggling array of lithologic, metamorphic and structural features, which can host an equally mind-boggling variety of mineral deposits and generate very complex patterns of geophysical anomalies (e.g., in northeastern Alberta, Langenberg and Nielsen, 1982; Langenberg, 1983; Sprenke et al., 1986). In areas with a polyphase deformational, igneous and metamorphic history, to construct a simple geophysical model may be impossible. Each locality and each deposit is different from another, necessitating detailed field mapping and varied geophysical surveys.
Seismic methods are designed mainly for stratified rocks with relatively uncomplicated structures. Mineral explorers rely on a wide range of geophysical techniques including magnetic, gravity, electrical, electromagnetic and radiometric, and above all on strong knowledge of hard-rock geology and on results of local field mapping and exploration drilling.
By itself, no geophysical anomaly can simply be correlated with lithology (Lyatsky, 2004). Instead, anomalies arise due to variations in some specific physical properties of rocks. These physical properties are a function of the rocks’ entire history as well as their present state. By itself, an anomaly says nothing about the nature, lithology or age of its rock source.
Reflection seismic surveys indicate changes in the acoustic impedance of rocks, especially where these changes occur across relatively flat-lying boundaries. Lateral variations in rock density give rise to gravity anomalies, and lateral variations in rock magnetization produce magnetic anomalies. Contrary to an occasional misconception, density and magnetization are rock properties quite independent of one another. Vertical changes in rock magnetization and density in a perfectly flat and layered earth would yield no magnetic or gravity anomalies, but these geophysical methods reveal lateral variations and high-angle discontinuities.
Survey and data-gridding parameters are chosen to resolve all expected useful anomaly wavelengths from the source under investigation, at minimal cost. At a relatively low cost compared to seismic surveys, gravity and magnetic techniques can provide geophysical coverage of large and small areas. In many Canadian regions, fairly sparse but useful magnetic and gravity grids are available at zero cost from the federal government. Calibrated with geological knowledge, gravity and magnetic anomalies can yield an indirect but extremely useful picture of lateral changes in rock composition and structural patterns.
Superb and readable guides to the basics of gravity and magnetic methods for non-specialists were created by Nettleton (1971) and Goodacre (org., 1986). More elaborate discussions of these and other geophysical techniques can be found in various texts, such as Telford et al. (1976). This latter book on my shelf is much beaten and dog-eared, having traveled to many field camps. A good collection volume on the uses of gravity and magnetic data in exploration and research, including case studies, was published by Hinze (ed., 1985). Along with my own experience and published work as well as the Canadian Geophysical Atlas (Geological Survey of Canada, 1990), these books form the basis for the discussion below.
Gravity Methods
Gravity keeps us on the ground. Without our planet’s gravitational attraction, we and everything else would fly off into space. Low gravity on the Moon allowed the Apollo astronauts to make their famously effortless high jumps.
Gravity readings on earth are not the same everywhere. The planet’s rotation and polar flattening are well known and easy to correct for, as are the small and predictable diurnal variations due to tidal forces of the Moon and the Sun. After these and other corrections, we are interested in map-scale gravity variations attributable to lateral changes in the density of local rocks.
Gravity anomalies in the Bouguer reduction, used commonly in land areas, take account of the Earth’s rotation, polar flattening, the recording field station’s latitude and elevation, and the gravitational attraction of the rocks below the station but above sea level. The terrain correction is applied in areas with non-flat topography.
More valuable for oil than mineral exploration, in marine and continental-margin regions the more elaborate isostatic and enhanced isostatic gravity reductions (Sobczak and Halpenny, 1990) often produce superb results. On a regional scale, useful results are sometimes obtained by applying a version of the Bouguer reduction offshore.
The gravity field is reassuringly simple, unipolar and almost perfectly vertical. The common unit of gravity measurement in exploration geophysics is milliGal (1,000 mGal = 1 Gal = 1 cm/s2 = 0.01 m/s2). These units refer to acceleration due to gravity, and the average value at the earth surface is around 980,000 mGal or 9.8 m/s2. By comparison, anomalies in mineral and oil exploration seldom exceed a few hundred milliGals.
Where the rocks underfoot are relatively dense and heavy, their extra gravitational attraction increases the downward pull and creates positive gravity anomalies (“gravity highs”). Where the rocks are light, the gravitational pull is diminished and the anomalies are negative (“gravity lows”). One can literally lose weight, if only very slightly, by moving from a gravity high to a low!
Airborne gravity surveys sacrifice some precision for rapid regional coverage; they are useful in frontier petroleum provinces where large block displacements are expected to cause big gravity anomalies. More commonly, gravity data are collected on the ground (Fig. 1) or (in offshore oil exploration) on board ships, by taking gravimeter readings from station to station or at a regular interval along ship tracks. In some land surveys, self-leveling gravimeters are briefly placed on the ground from a hovering helicopter, and then picked up and quickly moved to the next station for another reading.
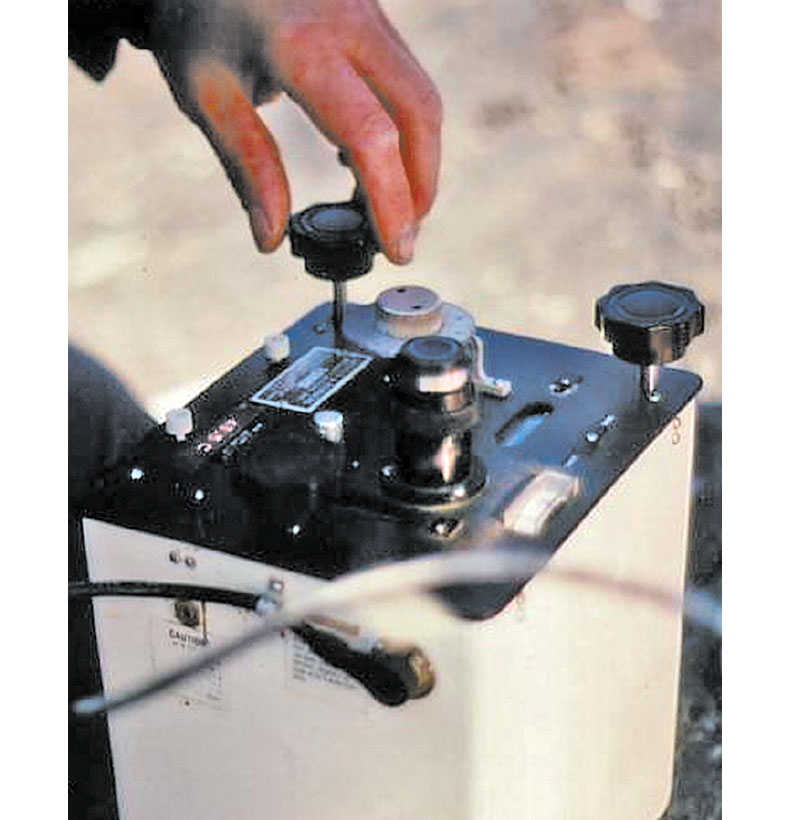
Magnetic Methods
Much more complicated are magnetic methods. Rapid and huge diurnal variations may arise unpredictably due to an extraterrestrial, solar wind of charged particles. The magnetic field itself is dipolar and usually non-vertical. Rocks can be magnetized in a vast and unpredictable variety of ways, induced or remanent, primary or secondary. Magnetization can be altered and lost when rocks are heated, reacquired when rocks cool, and created, destroyed or changed due to chemical alteration and other processes.
Certain minerals whose distribution can bear little relation to bulk lithologic patterns are the usual carriers of rock magnetization, whose lateral variations cause magnetic anomalies. The complexity of the magnetic field and of its anomaly-lithology relationships often complicates interpretation. Even a simple rock source can generate indecipherably complex anomalies.
A familiar use of magnetic data in Alberta Basin oil exploration is to delineate brittle faults in the crystalline basement (e.g., Lyatsky et al., 2005). Much more common is the use of magnetic surveys in mineral exploration.
Although land magnetic surveys are sometimes made locally, more usually the surveys are flown with fixed-wing aircraft or helicopters, with recording instruments in fixed external attachments (Fig. 2) or in slung “birds” (Fig. 3). Helicopters can provide quick and easy access to remote survey areas, and their maneuverability is well-suited for small-scale surveys. To examine the distribution of several rock properties at once, and because electrically conductive ores are sometimes also magnetic, aeromagnetic data over mining properties are commonly flown in conjunction with other geophysical surveys.
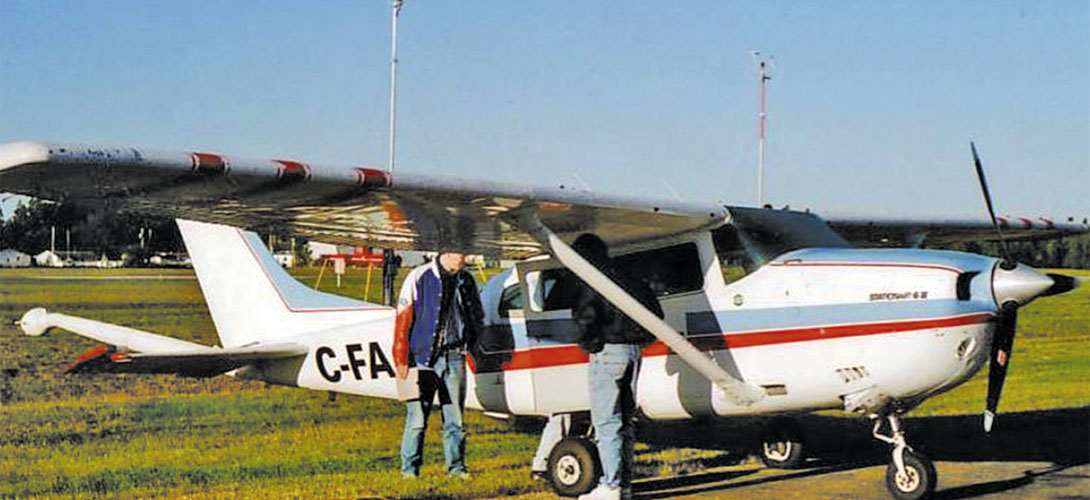
Magnetic-data preparation includes the removal of independently recorded diurnal changes and of the regional geomagnetic field. Particular attention must be paid to data “leveling” to avoid apparent corrugation along flight lines.
Detrital and chemically precipitated magnetic minerals are sometimes found in sedimentary rocks, but such minerals tend to be more common in extrusive and intrusive igneous rocks or in rocks that were metamorphosed. Distribution and internal zoning of plutons and volcanics, configurations of metamorphic zones, and structural patterns in crystalline rocks can be vividly reflected in magnetic maps.
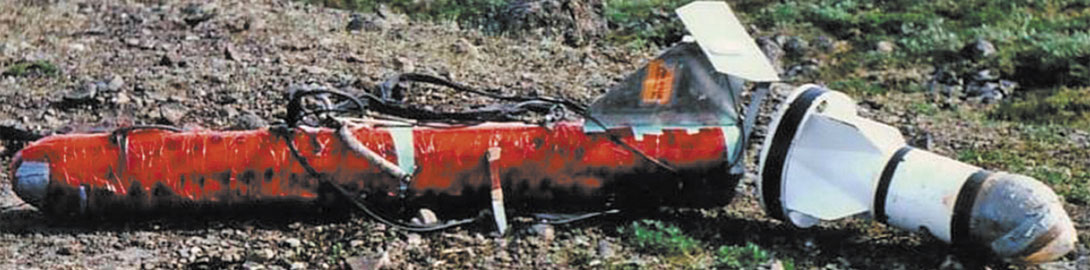
Some applications
Much of the mineral prospecting in Canada is conducted by junior companies relying on fickle equity financing in unstable capital markets. Northern climate severely limits the time available for field work (Figs. 4, 5) and the need for air supply in remote regions multiplies the costs astronomically. Stockexchange and land-use rules create additional limitations and costs. Speed and bang for the buck are of the essence in mining geophysical surveys.
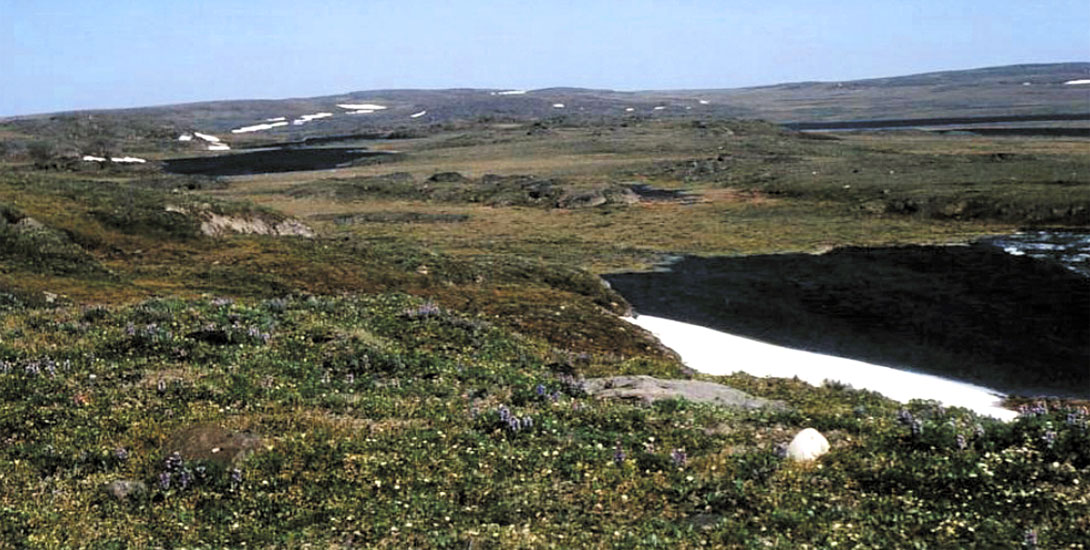
Magnetic methods are more popular in mineral exploration than gravity, not least because magnetic data can be quickly recorded from the air and in conjunction with other geophysical surveys. Land gravity surveys, by contrast, may require greater field efforts (Figs. 1, 5 and 6), more time, and more commitment of scarce capital. Besides, metal ores can be magnetic as well as electrically conductive, whereas high density of host rocks or limited deposit volume may leave ore deposits without clear gravity signatures.

Ductile and brittle structural patterns can greatly affect the location of mineralized zones. As in oil exploration (e.g., Babcock, 1973, 1974; Edwards et al., 1998; Lyatsky et al., 2005), of significant interest can be the distribution and age of brittle faults and fractures, capable of conducting mineral-bearing fluids from which ore deposits are sometimes precipitated.
As in oil exploration, such faults are sometimes identifiable from magnetic and gravity lineaments. Where brittle faults with subtle geophysical signatures are a target, data processing and interpretation may be similar regardless of whether the search is for hydrocarbon or mineral deposits. Faults juxtaposing dissimilar rocks can create density contrasts detectable in gravity maps, but gravity data are not always available in mining properties. Combined with surface geologic mapping where bedrock is visible through the till, fault detection often relies on identification of magnetic lineaments.
Ductile shear zones, igneous rock bodies, metamorphic and structural fabrics, and even protolith discontinuities can also generate individual magnetic lineaments or impart a grain to an entire anomaly domain. Such elongated anomalies may be hard to separate from lineaments due to brittle faults, especially if they are aligned. Brittle faults and fractures may have strong magnetic signatures if they are mineralized or contain dikes, but often their signatures are subtle. Sometimes they cut through the prevailing structural and anomaly grain. Even small fractures may be important: in northern Saskatchewan (Tremblay, 1972; Loutitt, 2008), it is sometimes not major faults but their secondand third-order splays and conjugate fractures that are associated with uranium mineralization.
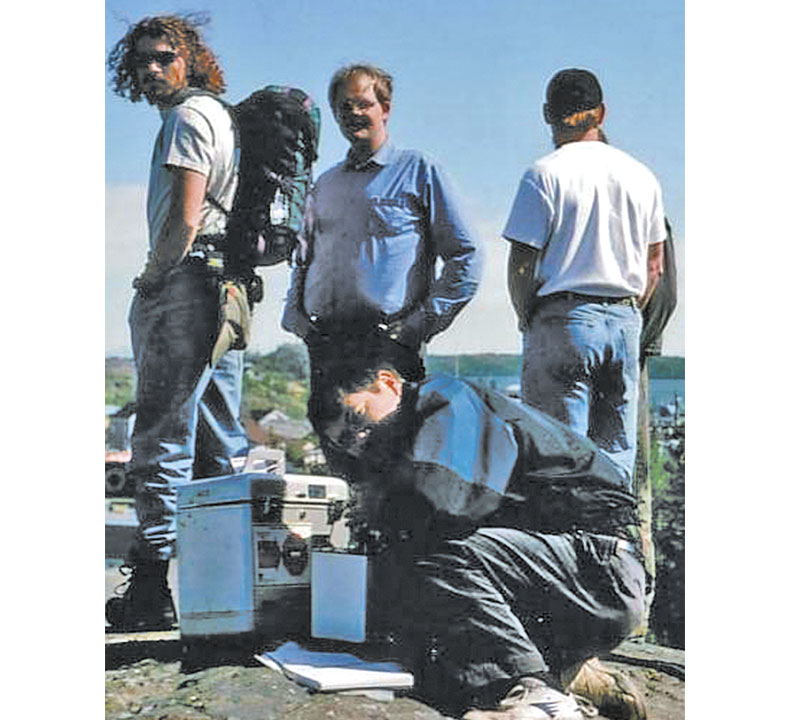
Data processing and interpretation to detect faults
Proper data processing is designed to highlight the anomalies of geological interest in a particular map area, and it involves much experimentation because it may be hard to know in advance which anomalyenhancement methods and parameters will yield the most useful results. Generically processed map products sometimes delivered by geophysical contractors are not always optimal for specific exploration targets.
Lineament-like artifacts can be created by poor data processing. In particular, it is desirable to minimize the use bandpass wavelength filtering (convolution filters are preferable), because linear artifacts may arise from Gibbs ringing. The best practice is to keep processing to a minimum, to avoid fancy “blackbox” techniques, and to rely on mathematically simple and intuitive procedures. As much as possible, enhanced anomalies should be easy to relate back to the original anomaly shapes. Enhancement of small, low-amplitude and shortwavelength anomalies generally helps to detect subtle lineaments.
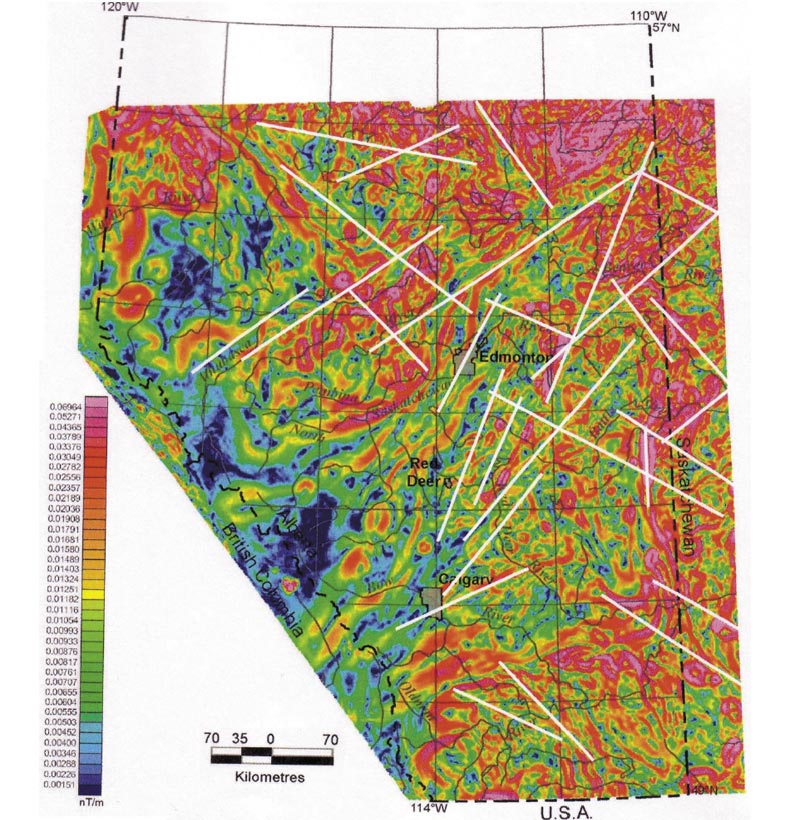
Good results are often achieved with horizontalgradient (Fig. 7) and vertical-derivative maps, sometimes in the second order. Horizontal-gradient vector maps (Fig. 8; Lyatsky et al., 1991; Lyatsky and Dietrich, 1998; Edwards et al., 1998) help to resolve details of anomaly clusters, but have a drawback if plotted gradient vectors themselves are long enough to obscure the desirable anomalies. Total-gradient (analytic-signal) maps, which somewhat artificially combine the dissimilar horizontal and vertical gradients, help to reveal the anomaly texture in potential-field maps and to highlight discontinuities in the anomaly pattern.
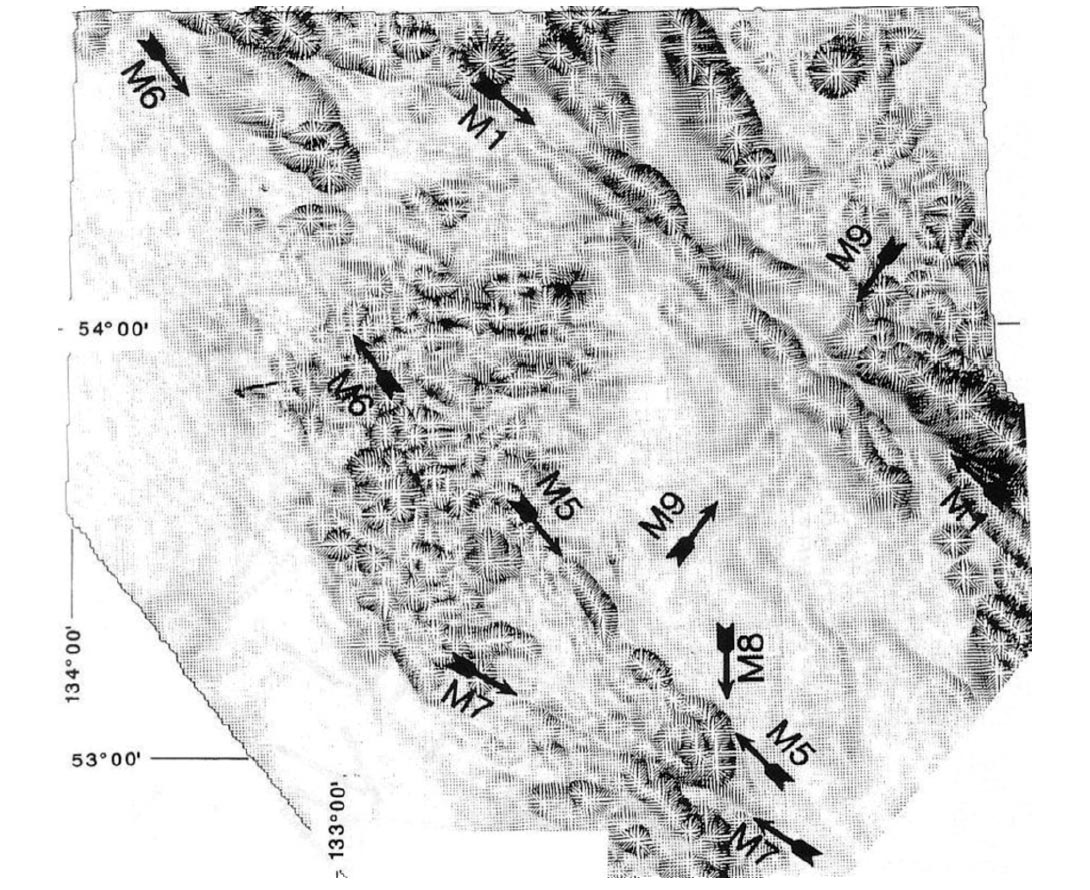
To make vivid local anomaly details, automatic gain control boosts amplitudes in areas with low anomaly relief. Separation of local from regional anomalies, avoiding wavelength filters, can be achieved by fitting to the data a third-order best-fit surface.
Very valuable are shaded-relief maps or shadowgrams (Fig. 9). This procedure treats a potential-field map as a relief and computes the shadow pattern that would be created if this relief were illuminated by the sun from a user-specified angle.
Particularly emphasized are the overall anomaly texture and individual subtle anomalies. For maximum utility, many shadowgrams are normally created for the same data set, with various “sun angles”.
Unless the maps are crowded, combining color and contour displays can greatly sharpen the depiction of anomalies. Geophysical lineaments, desirable in a hunt for faults, are best picked by hand, by placing a plotted potential-field map on a table and viewing it at a low angle from many different directions. A lineament can be a straight gradient zone, a linear anomaly, a break in the dominant anomaly pattern, or an alignment of several local anomalies. As with any geophysical interpretation, magnetic and gravity lineaments cannot be verified as faults without geological ground-truthing.
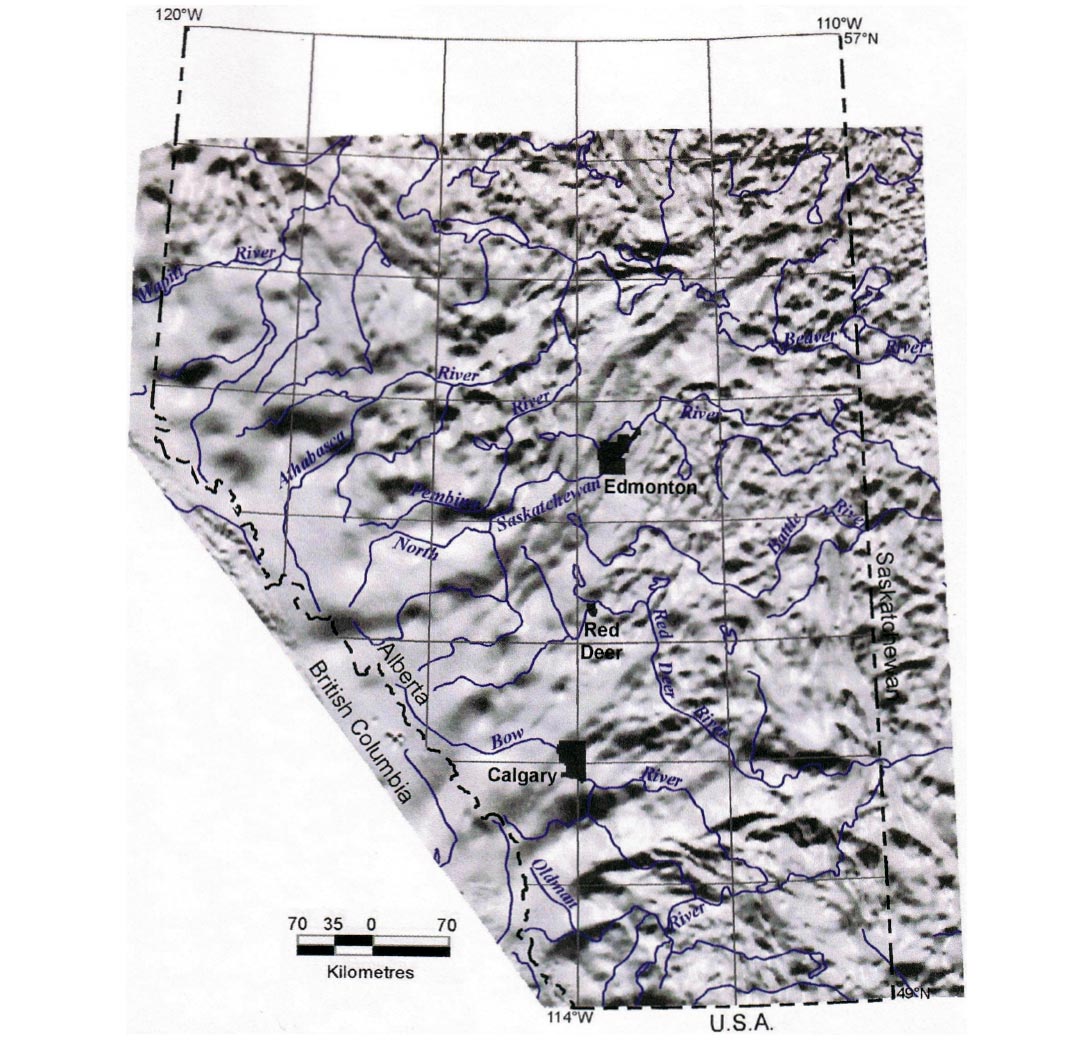
Diversifying Geophysical Education
Many geophysical methods yield fruitful results by exploring different physical properties of rocks (Fig. 10). Focusing college curricula on just some of those techniques to the virtual exclusion of others limits the young graduates’ career choices, and it reduces worker pools for employers. Avoiding early soft-rock or hard-rock specialization, undergraduate geophysics programs should produce well-rounded professionals able to operate in a wide variety of exploration environments and circumstances.
My own career has been greatly enhanced by acquiring skills, including in gravity and magnetic methods, marketable in both oil and mineral exploration and even in earthquake forecasting and political analysis. This has opened the doors to some magnificent field work and many fascinating projects (Lyatsky, 1996, 2006, 2009; Enachescu et al., 2009).
Excessive specialization in one set of extractable commodities or exploration techniques is a poor service to students, employers and taxpayers in the long run. Local business and professional groups are raising their voices to diversity college curricula (Babin, 2010). For the good of us all, may this call ring clear!
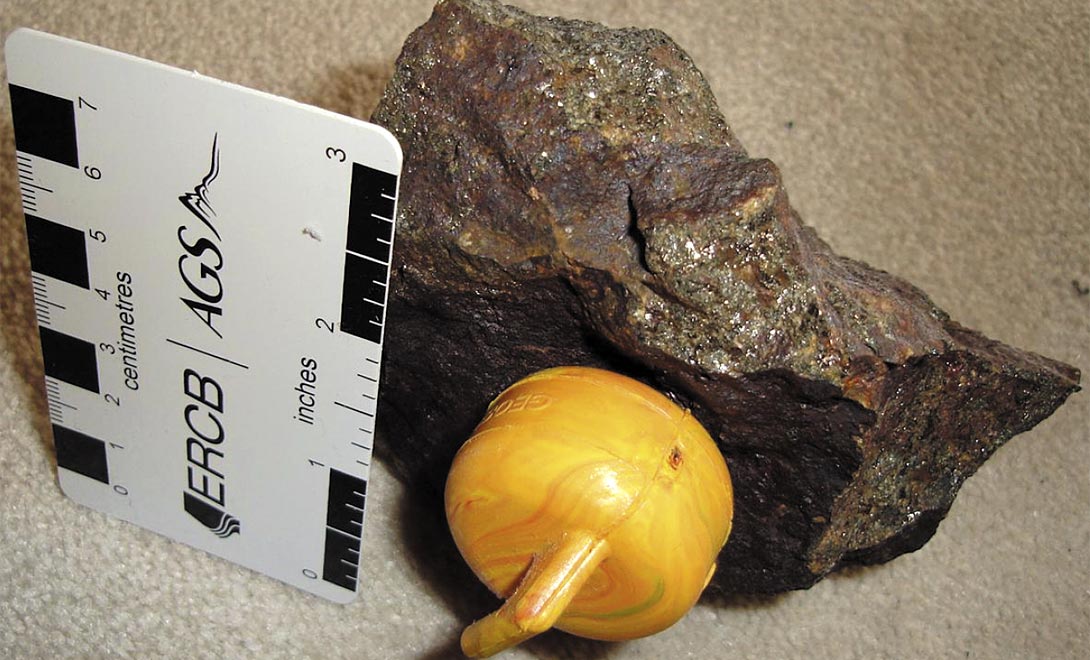
Join the Conversation
Interested in starting, or contributing to a conversation about an article or issue of the RECORDER? Join our CSEG LinkedIn Group.
Share This Article