Introduction
Like the oil and gas industry, the mining industry is a cornerstone of the Canadian economy, with production of non-fuel minerals in the order of $12 billion annually. With a few notable exceptions (e.g., the spectacular Voisey Bay nickel-cobalt discovery in Labrador), the Canadian mining industry is looking progressively deeper for new ore deposits to replace declining reserves at existing mines. This requirement for deep exploration is driving the search for new technologies to reduce costs and increase drilling success rates.
Traditional geophysical exploration methods in the mining industry have relied heavily on potential-field and electromagnetic methods, both airborne and ground-based, to locate drilling targets. Although it is generally recognized that seismic methods provide sufficient depth penetration and resolving power for deep exploration, the prevailing view of the mining industry is that seismic profiling is cost ineffective. Often cited are the paucity of strong, unambiguous reflections that typify a sedimentary basin, as well as a general lack of understanding of how observed signals correlate with subsurface features. Recent research may alter this view, pointing toward a possible new niche for the seismic exploration industry in Canada.
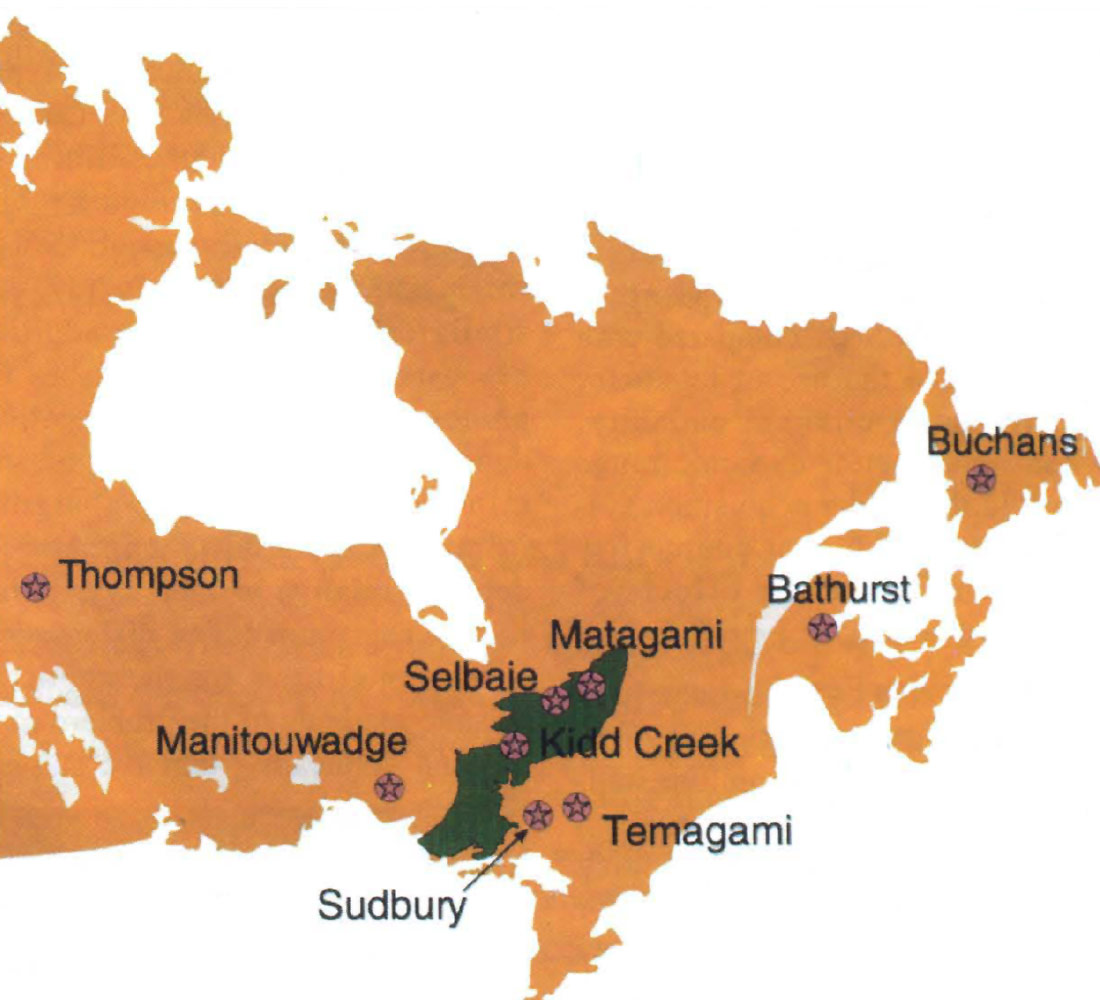
Lithoprobe
Since 1990, the Geological Survey of Canada, in collaboration with industry, has undertaken a systematic program to evaluate the use of seismic-reflection methods for mineral exploration. This endeavour was initiated by Lithoprobe seismic surveys designed to investigate large-scale crustal structures in Ontario and Quebec. Several of the Lithoprobe profiles passed through important mineral producing regions in the Abitibi subprovince (Fig. 1). With partial funding by industry, several seismic profiles were recorded using high-frequency parameters (20-m source and receiver spacing, 30140 Hz sweeps, 240 channels, 120-fold coverage, 2-ms sample interval, 5-s correlated record length with a 12-s sweep) to complement the regional Lithoprobe lines. The results of this work indicate that volcano- plutonic rocks of this region yield prominent reflections that can be correlated over significant distances (Clowes, 1994). Figure 2 shows migrated seismic sections from Matagami, Quebec that exemplify the reflectivity of upper-crustal rocks in the Abitibi belt.
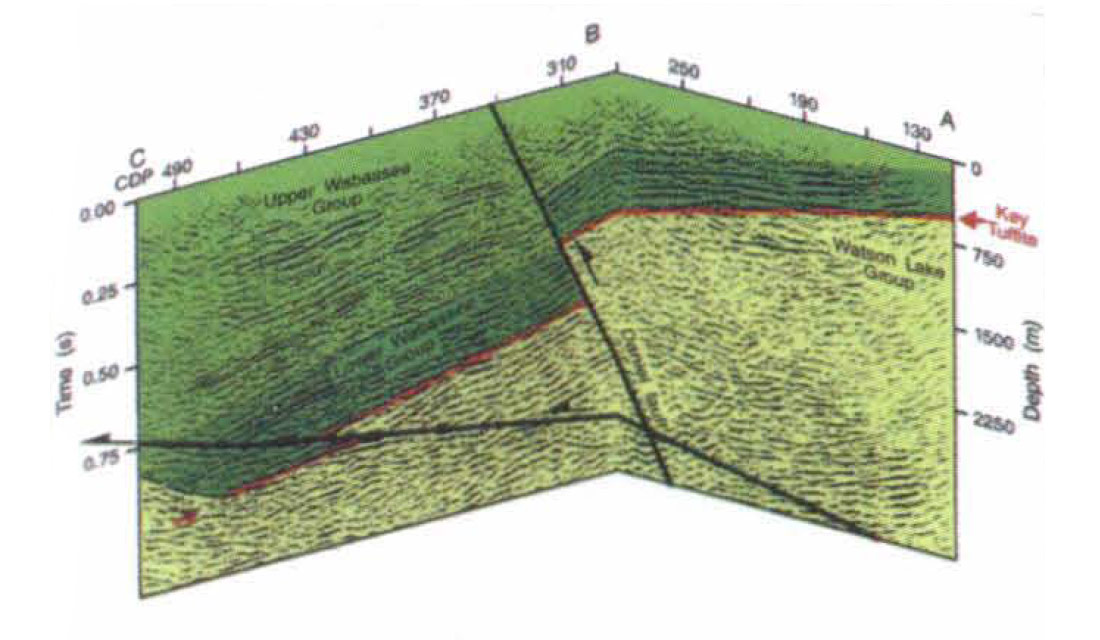
The Lithoprobe surveys provided the mining community with generally better images than what had been observed in the past (e.g., Reed, 1993). We attribute improvements in the SNR compared with previous surveys to the use of telemetry systems with large channel capacity, which yield much better dynamic range and higher fold than older acquisition system , and the use of higher source frequencies that mitigate the effects of source-generated noise.
Physical Rock Properties
One of the first questions to arise from the Lithoprobe high-resolution studies was "What is the cause of the observed reflections?". Since boreholes for mining exploration are not routinely logged for acoustic velocity and density, the data necessary to answer this question was not available in 1990. This prompted laboratory and in situ borehole logging studies of physical properties of crystalline host rocks and massive sulphide ore deposits. Since then, hundred of samples have been analyzed in the laboratory and approximately 20 boreholes have been logged for acoustic velocity and density.
The key results of this work (see Fig. 3) can be summarized as follows: I) as expected, velocities and densities of silicate rocks are tightly clustered around the well known Nafe-Drake curve; 2) for a given rock velocity, massive sulphide ore deposits are more dense than rocks that fall on the Nafe-Drake curve; and, 3) the acoustic properties of a composite ore material consisting of several sulphide minerals and a host silicate rock are governed by simple mixing laws (Salisbury et aI., 1996). Many of the mining camps studied are characterized by bimodal sequences of felsic and mafic igneous rocks, which produce inherently reflective stratigraphic packages. In addition, the key parameter that distinguishes the sulphide minerals from typical silicates is density; the greater density of sulphide minerals can produce a significant increase in the acoustic impedance of ore deposits relative to their crystalline host rocks. This impedance difference is the fundamental basis for the application of seismic methods to the problem of detecting and delineating massive sulphide ore deposits.
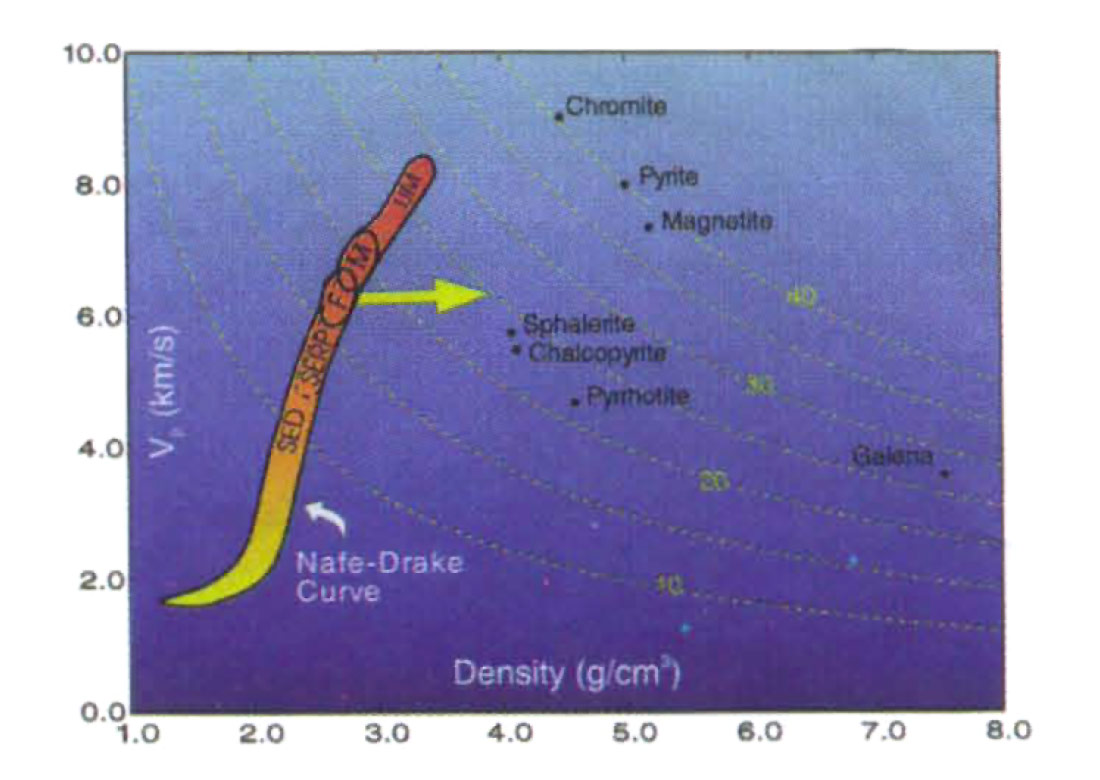
VSP studies
To verify the reflectivity of key lithologic contacts at seismic frequencies, a number of vertical-seismic profiling (VSP) studies have been conducted at representative locations on the Canadian Shield. These studies have made use of three-component receivers and have, in general, confirmed predictions based on measurements of acoustic rock properties by showing that both ore deposits and stratigraphic contacts produce reflections (Eaton et aI., 1996). The VSP studies have also demonstrated the important (but often neglected) contribution of S waves to the wavefield, since the Q-factor for both P waves and S waves in crystalline rocks is very high.
Hybrid "side-scanning" VSP surveys have also been acquired in cases where stratigraphic contacts are near-vertical (a common situation in Archean greenstone belts), in which case surface seismic profiling would not be expected to yield useful results. Promising results from these initial surveys have prompted pinoff research efforts aimed at developing new downhole seismic techniques to image the rockmass surrounding a borehole.
Seismic processing
Our experience with the generally low SNR seismic data from hardrock terrains suggests a number of problem areas that require careful attention during data processing. Some of the most important processing steps include: refraction static correction, first-break and NMO stretch mute and the preservation of large source-receiver offset traces. Refraction static corrections, while always important, are particularly significant in the hardrock setting. The high velocity of basement rocks (>6 km/s) beneath a low-velocity overburden layer that vary in thickness between 0 and 100 m imply that unresolved statics can prevent any reflections from stacking in phase. Furthermore, because of the low SNR, most residual static correction algorithms perform poorly on this type of data. The complexity of shallow geological structures requires that special care is taken when muting the data, to ensure that the maximum fold is retained at the target level. Mute functions are generally based on the first-break pick time (i.e., a constant of 20-50 ms is added to the first-break picks to define the mute), while we tolerate large NMO stretch to allow data at early times and large source-receiver offset to contribute to the stacked section.
Synthetic modelling
Comparison of seismic images from hardrock terrains with typical seismic sections recorded in sedimentary basins indicates that the scale-length of reflectors in the former case is much smaller. In fact, the validity of classical geometricaloptics approximations often used for seismic modelling, which provides the intuitive basis for many interpretation and processing techniques, probably does not hold for frequencies of interest (20-200 Hz) in a typical hardrock setting. For this reason, we have used an alternative forward modelling approach based on the Born approximation, a technique that is valid for arbitrary inclusions in a smoothly varying medium, provided that the scattering is weak.
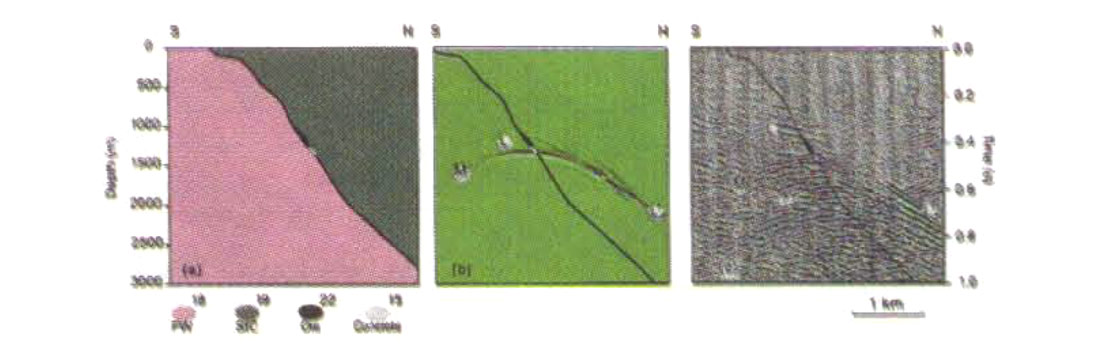
Synthetic and observed seismic data from the Creighton massive sulphide deposit in the Sudbury area are compared in figure 4, showing the contrast between the scattered signals from an intact ore body and a mined-out cavity. One of the key differences is the directional attributes of the scattering response. These and other modelling results suggest a strong tendency for lens-shaped deposits to direct scattered energy in the down-dip direction, whereas the largest amplitudes for scattering from open cavities comes near the apex of the diffraction (figure 4).
Quo vadis
These initial studies have culminated in several tests during 1995 and 1996 of 3-D seismic methods over known ore deposits (see cover picture). One of the main objectives of these pilot 3-D surveys was to establish templates for future reference. These datasets are currently being interpreted and have not yet been released for open presentation, but will be published in the literature in the near future to provide case histories for exploration.
Encouragement provided by the results of research to date may lead to further application of seismic methods by the mining industry in the coming years. To the seismic industry, this presents a tremendous opportunity for growth in a very large, untapped industry sector.
Acknowledgements
Funding for this research program was provided by the GSC, Falconbridge Ltd., Inco Ltd. and Noranda Mining and Exploration Ltd., through the GSC's Industrial Partners Program.
Join the Conversation
Interested in starting, or contributing to a conversation about an article or issue of the RECORDER? Join our CSEG LinkedIn Group.
Share This Article